Lithospheric foundering
Lithospheric foundering describes the idea that part of the lower crust or mantle lithosphere (the “plate” in plate tectonics) can become so dense that it detaches and sinks into the mantle. Over geologic time, the lithosphere behaves as a viscous material, and this process can be analyzed as a Rayleigh-Taylor instability.
Rayleigh-Taylor instabilies describe a wide range of phenomena, from stellar nebulae, to mushroom clouds, to a tablespoon of balsamic vinegar suspended atop a cup of olive oil. If plate tectonics describes how rigid tectonic plates move laterally, lithospheric foundering is one way to describe how hot, weak continental lithosphere deforms vertically. Rayleigh-Taylor “drips” could resemble giant raindrops, over 50 km wide, made of solid rock, that descend into the mantle within a few million years. These lithospheric drips would effect surficial geology, tectonic deformation, and melting. To test this idea, therefore, we look closely at sedimentary, volcanic, structural, and geophysical evidence. We also build numerical models in an attempt to predict such data from physics-based equations.
In a review of relevant literature, we found 26 locations on Earth for which researchers have proposed lithospheric dripping. Even well-known landscapes like the Grand Canyon may be partially generated by lithospheric dripping. We also synthesized numerical modeling studies to propose that lithospheric dripping occurs as two distinct types. The first type involves upper-crustal compression and basin formation; the second type involves upper-crustal extension and topographic uplift. The main factor that determines which type prevails is the strength of the crust relative to that of the mantle lithosphere, with strong crusts (>10x stronger than the lithosphere) favoring the former type, and weak crusts the latter. This conclusion is not totally new, as mathematical solutions of Rayleigh-Taylor drips have long recognized these two distinct outcomes. We found, however, that more complex geodynamic models also predict these two distinct outcomes (with some added complexities), and that both types of dripping should be found on Earth. This result suggests the second type of drip (weak crust), which has received relatively little attention by geologists, should be considered in locations where the crust is hot and weak.
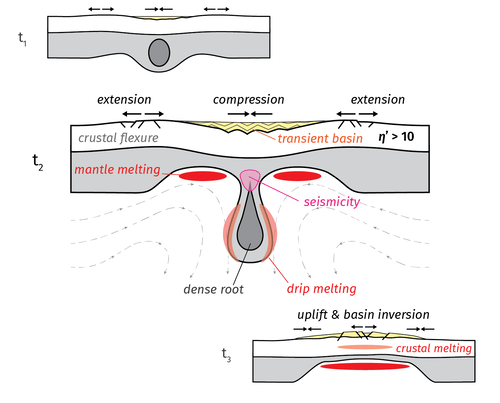
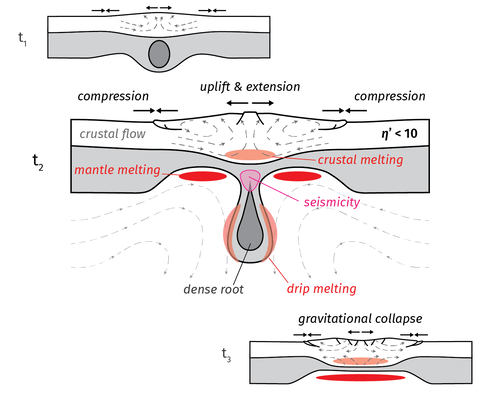
Illustration of strong-crust (top) and weak-crust dripping (bottom).
One such region of hot and weak crust is the Central Andes, Earth’s second-largest mountain belt. Evidence from the Southern Puna Plateau in the Central Andes suggests that the weak-crust type of dripping may have occurred during the Miocene. The “weak-crust drip” hypothesis difficult to test in practice, because relatively few geodynamic models have investigated the effects of dripping beneath a weak crust. We have relatively little understanding of the potential effects of a weak-crust drip. We know that they should cause upper-crustal extension above the drip, along with crustal thickening and perhaps partial melting (see the illustration, above). There are important outstanding questions, however: what happens after the drip detaches? Does the crust rebound by deforming compressionally, or does the thickened crust collapse under its own weight? What happens at the edges of the drip? Is compression strong enough to significantly deform upper-crustal rocks? What about if we impose a background of tectonic strain, say moderate compression due to oceanic slab subduction? These are all questions that apply to the purported drip in the Southern Puna Plateau, but that cannot be definitively answered by referencing existing geodynamic models.
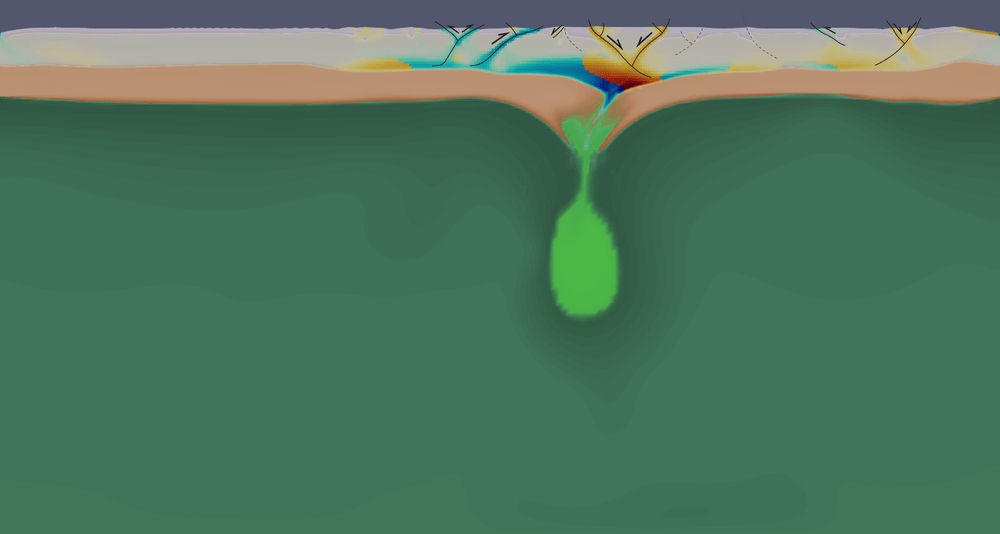
To address these questions, we recently submitted a paper of a numerical modelling study designed to explore these weak-crust drips more thoroughly, refining the dripping hypothesis and providing falsifiable predictions that can be compared to geologic data in the Southern Puna.
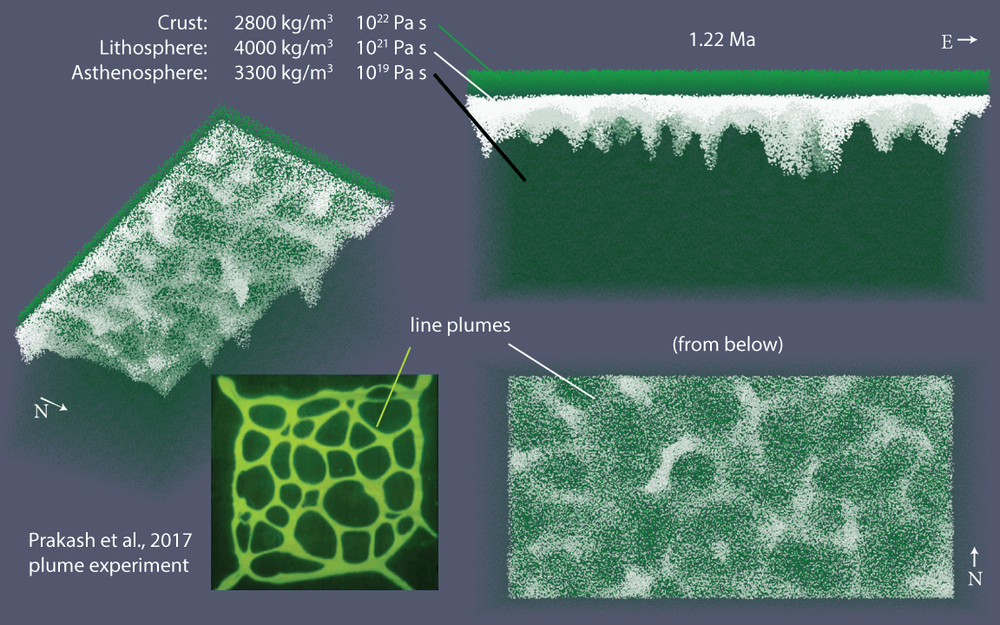
A very simplified three-dimensional model of lithospheric dripping (constant densities and Newtonian viscosities) compares favorably to a laboratory experiment of the Rayleigh-Taylor instability, recreating features such as “line” or “curtain” plumes, linear instabilities that connect the more significant “drip” downwellings. What this model and laboratory experiments lack are the strong gradients in viscosity and density that characterize Earth’s lithosphere.
Teasing apart the evidence for lithospheric dripping involves understanding the timing and kinematics of tectonic deformation, sedimentation, and volcanism in a region. The Antofalla region of the Southern Puna is situated near the center of an inferred dripping event (mainly based on patterns of basaltic volcanism, which pockmarked the landscape with cinder cones and flows), but the tectonic structures in the region had not been mapped in detail. Over two field seasons, we mapped the region, collected geochronology and thermochronology samples, and inferred episodes of tectonic deformation. In two papers published in Tectoncis, we present evidence for multiple episodes of shortening followed by an episode of transient, but significant, extension. This extension is especially significant because it created the Antofalla depression and ultimately the salt-flat landscape that we see today. We find that it occurred earlier than previously thought in this area (~16 Ma vs. only a few Ma) and was followed by a new episode of horizontal shortening, which is currently active in the region.
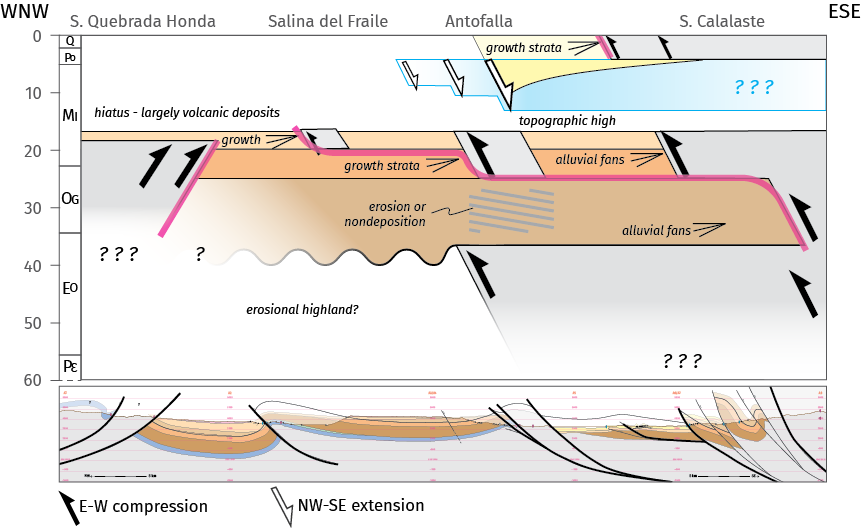
Summary of Cenozoic tectonic events in the Antofalla region (Puna Plateau) inferred from geologic mapping, cross-sections, and low-temperature thermochronology. Black arrows show the location and timing of compressional events. Pink lines trace the edge of compressional deformation as it propagates outward from the core of major mountain ranges (Quebrada Honda and Calalaste) into the sedimentary basin. The blue shape illustrates an episode of transient extension inferred from sedimentary relationships and low-temperature thermochronology.
One key challenge for anyone proposing lithospheric foundering is answering the question of how the lithosphere become dense enough to founder. If you are in a region with intense arc volcanism, one way to do this is to invoke the accumulation of dense mineral phases that result from differentiation of basaltic melts into granitic crusts. Minerals such as pyroxenes and garnets are thought to accumulate at the base of the magmatic batholith, and would founder after reaching a critical thickness. Another way is to metamorphose a swath of lower crust at high pressure. In retro-arc mountain belts, the second mechanism may be more relevant, and may be capable of producing the large Rayleigh-Taylor drips geologists discuss for the Andes. My work on eclogitization is an attempt to address this question from a thermodynamics perspective. The goal is to model chemical reactions that can transform lower crustal rocks into dense eclogites and trigger lithospheric dripping.